Frequently Asked Questions
General questions
on Renaissance Fusion
on IP, publishing and collaborations
on funding
FAQ on liquid metals (LM)
on LM benefits
on electromagnetic control
on materials and corrosion
on LM droplets

General questions
on Renaissance Fusion
-
Renaissance Fusion is a hardware R&D company with the goal of designing, building, and commercializing a fusion power-plant based on the stellarator, HTS and liquid metal technologies, on time to contribute solving the climate crisis caused by carbon emissions.
-
In 2019 our founder Francesco Volpe presented in New York and Milan the idea of creating the first magnetic fusion start-up in Europe. In October 2019 he visited 5 candidate cities in France, the Netherlands and Spain. In 2020 he moved to Grenoble, France, where in July he officially registered the company.
Francesco chose to create a private start-up (not spun off from any university or public laboratory) to offer an alternative path to fusion: imbued with scientific rigor and academic collaborations, and yet fully relying on the agility and speed of a start-up.
-
We are a single, multi-disciplinary company organized in 5 teams developing 3 technologies: one team on HTS film deposition, one on liquid metals and three on fusion science and engineering. The 5 departments share business, admin and technical resources, and frequently discuss and exchange with one another, for good reasons: far from being a random collection of good ideas, the three techs are highly synergistic to each other. To make an example, HTS generate strong magnetic fields that facilitate the levitation of current-carrying liquid metals; at the same time, thick Li-based liquid walls shield the delicate HTS from damage by fusion neutrons.
Spin-offs on HTS or liquid metals are not excluded in the future, but for now and for the foreseeable future we are fully dedicated to the holistic project.
on IP, publishing and collaborations
-
We are self-aware dwarfs on giants’ shoulders, thankful for the body of knowledge published by public labs over the decades, eager to “give back” and jointly advance fusion. For this, we regularly present our results at conferences, we have published or recently submitted several journal articles, and we welcome scrutiny by the scientific community. At the same time, to protect some findings from unauthorized commercial exploitation, we ask our scientists and engineers to “patent first, publish later” (or to be precise, submit the patent application first, then the paper -without waiting for the patent to be granted). The good news is that patent applications are much faster to write, and no scientific paper has been delayed in this process.
-
We already have several collaborations in Europe and the USA (see https://renfusion.eu/people) and are constantly on the look for more collaborations with public organizations and, on selected topics, with private companies. The only criteria are that collaborations be mutually beneficial, and that timescales, cultures and risk-profiles are reasonably well-aligned. Fair warning: we strive to be fast and explore unbeaten paths, with the result that either we will publish a major paper together, or none. Steady streams of incremental papers are not our forte. Other than that, sky is the limit and there are more and more grant opportunities for public-private partnerships. If you have an idea for a collaboration, send us an email at contact@renfusion.eu !
-
There are several formats:
— Informal collaboration: each organization pays for its scientists/engineers to realize a design, simulation, or experiment, or build an instrument or device of common interest. Ideally, the organizations will complement each other’s strengths. The topics, a rough roadmap and division of roles can be agreed in a memorandum of understanding of a couple of pages – we like to keep things simple.
— RF and the French government, through the CIFRE program, can co-sponsor a PhD thesis at a French university.
— RF and a university, anywhere in the world, can co-sponsor a PhD thesis or post-doc.
— Joint grant application to a French, Swiss or European program admitting or even encouraging public-private partnerships. As a start-up, it might be a win-win if we partner with universities, national and international labs, and select large companies. For international applications, as a French- and Swiss-based organization, it might be good to partner with organizations in other countries.
on funding
-
We are a pre-revenue company at the product development stage, primarily financed by external funds. After an Angel round in June 2021, we closed a Seed round in May 2022 with several international VCs, for a total of approximately 16 M€. Our investors include Lowercarbon Capital, Positron Ventures, Norrsken VC, HCVC, Unruly Capital, Excellis, and Exor Ventures. In addition, we received small grants from BPI France in 2020 (“Bourse French Tech Emergence”) and from the INFUSE program in the USA, in 2021-22. In November 2023 we were awarded a 10 M€ grant in response to the Call for Proposals “Innovative Nuclear Reactors” organized by BPI France in the framework of the France 2030 program. As part of the award we will also receive “in kind” help from CEA institutes in Cadarache, Grenoble and Saclay, worth several millions. That was phase 1 of a 3-phase program. Grants of up to 80 M€ and 300 M€, respectively, will be made available in phase 2 and 3.
-
At the moment we have no EU projects running, but we look forward to forming consortia with universities, national labs and industrial partners in 2-3 EU countries and jointly applying for funding. We are especially interested in complementary skills and technologies. Together we will bring HTS, liquid metal and fusion inventions closer to market and societal impact. If interested, reach out at contact@renfusion.eu.
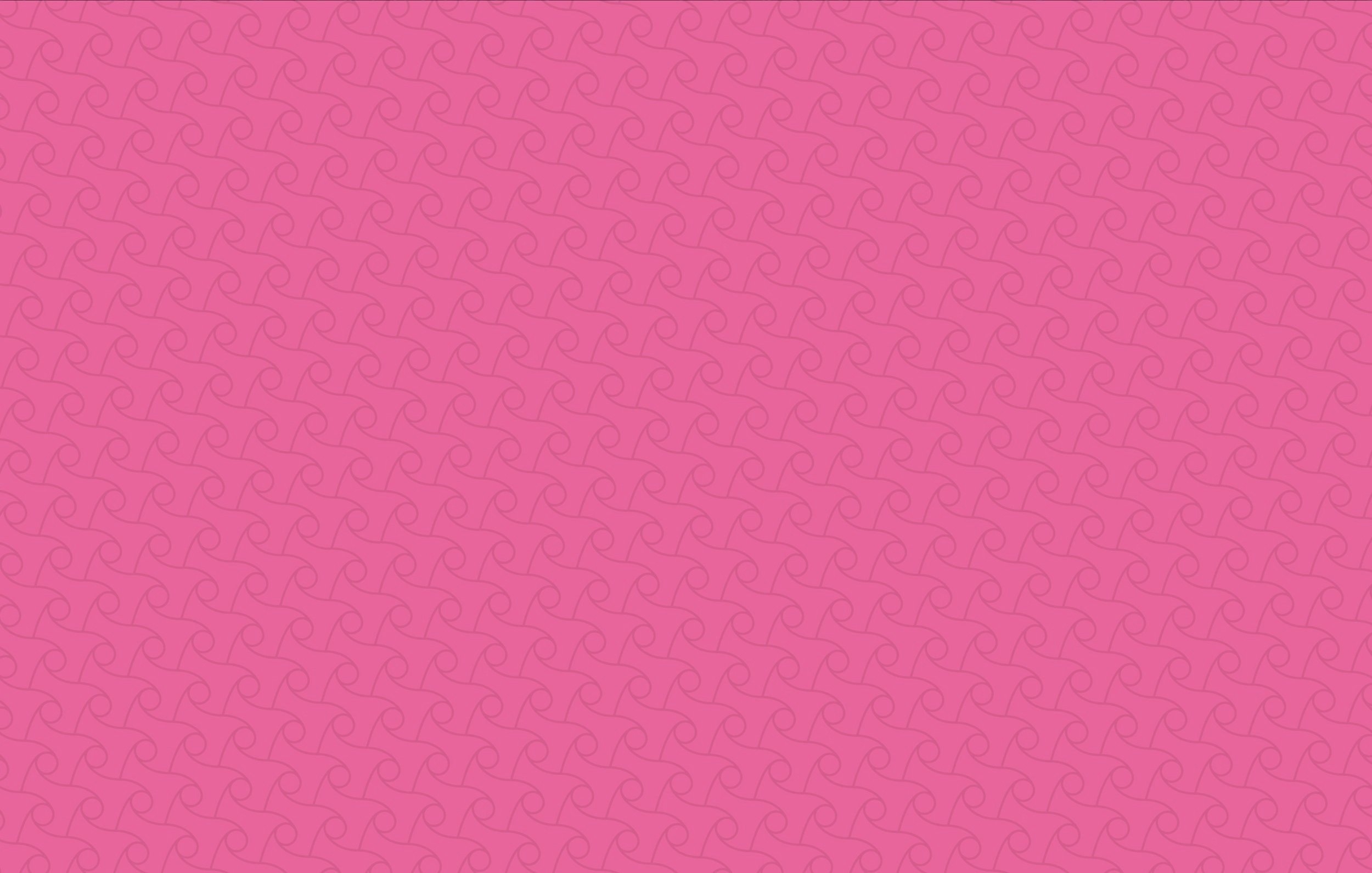
Fusion FAQ
-
For 40 years fusion actually over-delivered: from the 50’s to the 90’s, a key performance metric called “triple product” grew by 6 orders of magnitude. It is now within 1 order of magnitude from net fusion power.
The problem came afterwards: since the late 90’s, while physics understanding kept improving dramatically, performance stagnated. The reason is that the construction of next-step devices of higher performance had stagnated. Fusion devices had grown so large and expensive that they became an experiment in geopolitics, public funding, megaproject management and construction engineering. Delays were accumulated for W7-X and ITER.
-
In general, four things:
1. The commercial availability (and start-up adoption) of High Temperature Superconductors enabled 2x stronger magnetic fields, hence 8-16x smaller fusion-devices. Smaller is cheaper, although not by 8-16x.
2. Traditionally cautious scientists moved from national labs to startups and embraced their faster pace, bigger risks, frequent iterations, and milestone-based approach.
3. Risk diversification by private investors encouraged “more shots at targets”, new approaches, or new takes at old ones. Statistically, 2-3 of them could achieve low-cost fusion electricity. By contrast, public funding had converged on few large devices for few established approaches.
4. Climate change: the public and investors are more concerned, better informed, and they recognize the need for a portfolio of renewables, energy storage and baseload sources. At the same time, they understand that hydroelectric has been used to capacity and has its own impact; deep drilling for geothermal has not been confirmed; traditional nuclear lacks public acceptance and many countries are moving away from it. Fusion, the most exotic of all baseloads, is not so far-fetched after all: the 2050-60’s if you listen to public forecasts ‘a la NASA, but the 2030’s if you trust private start-ups ‘a la SpaceX.
on scale-up (NEW - Aug. 2024)
-
Fusion is the most fuel-efficient and resource-efficient energy source, and one of the most land-efficient. Therefore, there is practically no limit on the maximum number of fusion power-plants that can be built, from a natural resource perspective. There is no economic limit either, thanks to the geographic versatility of fusion (other energy sources are more site-dependent and become unprofitable in regions with limited wind, sunlight or land). Regarding fuel, deuterium and lithium will last for millions of years.
Rather than “how much”, the question is “how fast” fusion can scale to a sizable share of the global energy market. To other energy sources it took about two decades, but solar was remarkably fast: it “took off” around 2009 and then regularly outperformed all predictions by large margins, reaching a 5% market share a mere 14 years later, in 2023. Four trademarks made solar fast: simplicity of installation, no need for customization, low cost, low maintenance. To emulate that feat, at Renaissance Fusion we modularized our stellarator: self-contained, all-inclusive modules will be mass-produced at low cost in few centralized factories, shipped to the power-plant sites, and juxtaposed to one another to form the reactor. Liquid metals keep reactor part replacements and maintenance to a minimum. So yes, Renaissance Fusion is poised to scale as quickly as solar energy and provide abundant clean energy anywhere, anytime.
-
Cheap fusion electricity will at first replace coal, gas and other carbon-intensive, non-renewable energy sources. The environmental impact of this transition will obviously be very positive.
We predict that fusion electricity will be cheaper than wind and competitive with solar, but with the added benefits of 24/7 availability and high “energy density”. Hence, a gradual shift of the energy mix to more fusion will reduce material consumption, mining and the power-plant footprint, and land utilization - land that can be used for natural preservation or reforestation. Other good outcomes of cheap electricity include more recycling, desalination, access to drinking water, irrigation, access to electricity in developing countries, reduced geopolitical tensions over access to energy and related resources.
Ultra-cheap electricity can, however, also have bad outcomes: more consumerism, more waste, more power-plants, more mining to build them. This is known by economists as the Jevons paradox and by energy economists as the Khazzoom–Brookes postulate. Fortunately, if not even cheap solar energy has triggered such paradoxical effects, neither can fusion (fusion is predicted to become as cheap as solar, but not significantly cheaper; its advantages will rather be reduced utilization of land and resources, and 24/7 availability).
Should energy ever become as cheap as to trigger the Jevons paradox, then the availability of other resources, such as minerals, will play a bigger role. At that point, governments will probably have to implement constraining policies and special taxes or disincentives.
on Fusion Power Plants (FPP) (NEW - Aug. 2024)
-
Heat from the fusion reactions is mostly carried by neutrons and captured by our plasma-facing liquid metal wall. This first fluid transfers the heat to supercritical CO2 and finally to water. Heat is converted into electricity using an optimized turbine power conversion plant -in our case a Brayton-Rankine combined cycle plant achieving up to 51% efficiency. Additional details can be found in this publication: F.R. Famà et al., "An optimized power conversion system for a stellarator-based nuclear fusion power plant." Energy Conversion and Management 276 (2023): 116572.s
-
About 100. Unlike tokamaks, stellarators are steady-state and highly MHD-stable, hence the Plasma Control System (PCS) is greatly simplified and only requires few human operators. The Torus Hall is also highly automated.
-
With thousands of power plants, fusion will create hundreds of thousands of jobs. New jobs will be created in related industries upstream and downstream of fusion, including but not limited to metallurgy, cryogenics, vacuum technology, electrical engineering, sensors, microwave sources for plasma heating, electricity distribution.
-
A fusion power plant site can be installed almost anywhere, as we strive for small-sized high-field compact reactors with little land use. There are just a few basic criteria for safety and access to water that the site should meet. The selection of the ITER site was a great lesson for FPP sites. Interestingly, seismicity was not a showstopper.
on the relation with ITER & other start-ups
-
Not at all! On the contrary, we are dwarfs on giants’ shoulders, thankful to public laboratories for decades of fusion research. As a result of that, ITER is on the verge of proving the scientific feasibility of net fusion heat. In parallel, times are mature for startups like Renaissance Fusion to address the economic attractiveness of fusion. The missions are complementary. The strengths are complementary too: start-ups cannot do it alone, they need collaborations with and validation by the scientific community; public research, on the other hand, can benefit from the frequent, rapid iterations of start-ups, and from their agility in adopting emerging technologies such as HTS, which would not have been possible at ITER.
-
Q denotes the “fusion gain”, and Q = 10 means that the reactor produces 10x more heat than it consumes to keep the plasma hot. ITER has a high probability to reach this important milestone in the late 2030’s. In comparison, start-ups take a potentially faster but more uncertain path. Individually considered, their probability of success is lower than ITER’s, and the year in which they will attain Q = 10, if ever, is more uncertain. The ensemble of all most promising fusion start-ups, though, has a serious chance that 2-3 of them will reach Q = 10 before ITER. But again, this is probability. As humans, it makes sense that we place multiple bets on fusion, including a safe one like ITER.
-
Theranos was built upon the vision, delusion and, unfortunately, fraud by a single founder without deep technical foundations. By contrast, fusion benefits from over 70 years of scientific experimentation and progress. Public institutes regularly peer-review, cross-check and reproduce each other’s results. More than 40 startups are now developing prototype reactors to commercialize fusion, and while it is relatively easy to fake blood test results, it is much harder to fake the production of electricity or heat. That being said, like in any other emerging sector, some fusion companies will fail anyhow, not owing to fraud, but due to failure to retire all technical risks or to become cost-competitive. Some other fusion companies will specialize, some will pivot, and some will succeed.
-
We are most likely to succeed because we are reinventing the best fusion device (the stellarator – continuous, cheap electricity) and the most efficient fusion reaction (D-T) with the best/only solutions to the only three caveats of D-T stellarators:
1) Complex coil shape – Our solution to this is the best: our coils are subject to lower electromagnetic forces than competitors’ and are immune from the limitations of 3D metal printing.
2) Large size – Everybody wants to build HTS coils to generate stronger magnetic fields, resulting in smaller fusion devices. Our approach is the best: competitors buy scarce, pricey HTS tapes, whereas Renaissance Fusion is building machines to produce and sell its own HTS with a unique, wide form-factor that will instantly enable (i) large manufacturing volumes and (ii) a novel way to directly make laser-precise HTS coils. This will be faster, simpler, cheaper and more precise than winding, epoxying and positioning traditional HTS tapes.
3) Neutron-induced radioactivity and damage - We are the only magnetic fusion organization -whether public or private- with a solution to this. This is a unique competitive advantage, in a world where everybody, thanks to HTS (see point 2) is rushing to more compact fusion devices and Q>1 but seems to ignore the consequences for materials and economic feasibility. In compact reactors, the same amount of heat and neutrons will impinge on smaller surfaces, resulting in even faster damage and activation, and thus in more frequent maintenance and replacement.
Our solution is the most effective mix between invention, elegance, project feasibility and commercial attractiveness to bring fusion energy out of the lab and onto the grid.
on materials
-
We will use the deuterium-tritium fusion reaction because it has the highest “cross-section” (likelihood to occur) and requires the lowest temperature (we are still talking of more than 100 million °C!). Deuterium is abundant and easily extracted from ocean water, whereas we will breed our own tritium thanks to our unique lithium-based, liquid metal wall.
For a 1 GWe plant we will need approximately 220-330 Kg of externally sourced natural Lithium per year, and 100-140 kg of in-bred tritium per year.
-
Yes, there are approximately 30 kg of tritium reserves in the world, with annual production in the order of several kg. Far less than 1 kg of tritium suffices to start a fusion reactor. After being started, the power-plant will self-sufficiently produce the rest of the tritium that it needs. I fact, it will even produce a surplus of tritium, to start off additional reactors.
-
No: fusion needs for Lithium and rare earths are extremely small compared to the demand for Li-ion batteries, permanent magnets, and other markets. For example, a 1 GWe fusion power plant uses approximately 18- 28 kg of Li per month, whereas a single full electric car includes on average 10 kg of Li.
This is especially true for our reactor, which will rely on natural Lithium (7.5% Li6 and 92.5% Li7). Other fusion reactors will rely on enriched or pure Li6, which is 13x less abundant. Even so, there are proven resources in the order of 3 million tons. An additional 250 billion tons could be extracted from seawater.
on fusion compared to other energy sources
-
Fusion is the process of combining two light atomic nuclei to form a heavier nucleus, releasing a large amount of energy in the process. The most well-known fusion reaction occurs in the sun, where hydrogen nuclei combine to form helium.
Fusion products are not inherently radioactive in the same way as fission products. Fusion reactions primarily produce high-energy neutrons, which will bombard the surrounding materials and could make them radioactive.
The fusion fuel itself consists of two isotopes of hydrogen: one non-radioactive (deuterium) and the other radioactive but short-lived (tritium). Tritium’s half-life is 12.3 years. By contrast, fission produces highly radioactive byproducts, including isotopes of elements like cesium and iodine, which can persist for a long time. The radioactivity generated by fission can be a challenge for the handling and disposal of nuclear waste.
-
Energy scholars are clear: we need a diversified mix of (1) renewables, (2) energy storage and (3) carbon-free baseloads or, even better, load-following energy sources. That means energy sources whose supply can rapidly adjust, within minutes, to fluctuations in energy demand. The third ingredient will account for ~80% of the energy mix, and will feature three main contributors: hydroelectric, geothermal and nuclear, thereby including fusion.
Fusion should first replace fossil fuels (and fission, in countries where it is unpopular). We don’t think fusion should compete with renewables. On the contrary, we need “all hands on deck” to meet the daunting 2030 and 2050 goals of decarbonization and energy transition. In fact, through our HTS technology we are actually helping both renewables (more specifically, electricity generation and transmission from wind energy) and energy storage.When the climate crisis will be averted and fusion will be mature, though, it will become apparent that fusion is the densest energy source of all, requiring the least amount of land and mining of materials and having the lowest environmental impact, far lower than renewables. Simple math and economics will do the rest. We, and many others, predict fusion to become the dominant energy source globally during the second half of the century.
on the timeline
other questions on fusion
-
Machine learning and Artificial Intelligence (AI) have already played a major role in optimizing two tokamak experiments: real-time plasma shape control in TCV and real-time disruption prediction in DIII-D. In stellarators, various public and private groups, including Renaissance Fusion, started to use AI to find new stellarator shapes, for instance to better confine fusion-born alpha particles: confining them keeps the plasma hot and promotes further fusion reactions, without much need for external heating.

FAQ on Liquid Metals (LM)
on liquid metal walls’ benefits
-
Plasma-facing liquids can handle neutrons and energetic charged particles better than solid walls. For instance, they suffer no structural damage by neutrons, because they have no crystal structure. Erosion and sputtering are much reduced. Finally, the first wall can tolerate higher heat fluxes, if it flows (compared to a static solid wall with embedded cooling conduits). Important bonuses are reduced maintenance and more continuous operations.
-
Li-based liquid walls, even thin ones (< 1 mm), have benign erosion, sputtering and recycling properties. They basically act like sponges that absorb impurities and do not release them back in the plasma. This keeps the plasma clean, which is essential for fusion. One reason is the high chemical activity of Li -whether solid or liquid. Other reasons are physical (low sputtering and erosion) and they benefit from the wall being liquid.
-
Sufficiently thick walls (35 cm, for our choice of materials) stop most neutrons. This serves two purposes: preventing neutron damage and activation of the rest of the reactor, including HTS, and harvesting fusion energy, 80% of which is indeed carried by neutrons. The remaining 20%, in the form of alpha particles, remains in the plasma and keeps it fusion-hot.
Our Li-based wall is thick enough to breed all the Tritium (one of the fusion fuels) needed by the reactor. Effectively, our liquid wall is a “naked” breeding blanket, directly exposed to the plasma.
-
Flowing walls exhaust the extreme heat and particle fluxes from the plasma, thus increasing the lifetime of plasma-facing components.
In this approach, the flowing, plasma-facing liquid metal is the first working fluid of the power plant. In our specific design, heat is then transferred to supercritical CO2 and finally water.
on electromagnetic control
-
Liquid metals are electrically conductive. When an electric current flows through them, its combination with the ambient magnetic field used to confine the plasma generates a force, known as Lorentz force. Strategically placed electrodes result in currents and thus forces of proper direction, as to counteract gravity.
-
No: thanks to the high magnetic field generated by HTS to confine the plasma, the Lorentz force on the liquid metal can easily exceed gravity, even for small currents applied.
Said otherwise, parallel currents attract each other. In this case, the currents in the rigid HTS attract the deformable, current-carrying liquid metal. Since the attractive force scales like the current product, and currents in the HTS are very high (tens of MA), relatively low currents in the liquid metal suffice for levitation (few kA).
-
There will be sensors of thickness and velocity. In feedback with them, actuators will apply local currents, hence forces, and ultimately control the local thickness and velocity of the liquid metal. In fact, electrodes will act as both sensors and actuators, and will be aided by other types of sensors. An array of electrodes ensures good, uniform thickness. Experiments with arrays of sensors and arrays of actuators have already begun. Next, we will interface them and “close the feedback loop”.
-
Yes, there will be ports for plasma heating, diagnostics, pumping and pellet fueling. Initial experiments showed that the liquid metal just flows around the ports like a river flows around the pillars of a bridge: the flow is depleted in the wake of the port, and turbulence is enhanced. Both effects are slight; the former and, to some extent, the latter, can be electromagnetically counteracted by electrodes (see previous question).
on materials and corrosion (NEW - Aug. 2024)
-
The liquid metal will be in contact with solid parts both in-vessel and outside the reactor, in the plant that will clean it from impurities and other byproducts, extract heat, and extract tritium. For minimum maintenance and replacement, solid parts must thus have good resistance to corrosion by liquid metals. We deal with this by testing and implementing corrosion-resistant materials and coatings. Generally, other corrosion reduction strategies would be to reduce the temperature and flowrate. Those are not possible here, due to heat extraction from the reactor.
-
Instead of lithium or a lithium alloy, we will use a solution of lithium and lithium hydride. Since lithium hydride has a higher melting point (688 C) than lithium (180 C), the Li-LiH solution will have a lower tendency to evaporate. Consequently, it can operate at a much higher temperature than liquid Li (200 C higher), for the same evaporation rate. Note that the ultimate limit lies not in the evaporation rate “at the source” (the liquid wall), but in how much Li and H effectively reach the diverted plasma. This is a complex transport problem that we are currently modeling and will experimentally characterize and optimize. For now, based on peer-reviewed tolerable evaporation rates for Li, we estimate that the +200 C “advantage” will allow operations at ~700 C, possibly higher. The higher the temperature the merrier, for the thermodynamic efficiency of the reactor. Our goal is to approach 900 C as closely as we can, but even 700 C would be far hotter and far more efficient than any competitor.
on liquid metal droplets
-
In a tokamak, a liquid metal droplet or solid wall “flake” or dust cause a disruption: the stored energy and plasma current are lost in few ms. Its consequences (runaway electrons, halo currents, electromagnetic stresses etc.) can damage the tokamak. Interestingly, if a disruption is inevitable for other reasons, “killer pellets” or liquid jets can be deliberately injected to trigger the disruption in a controlled, mitigated manner.
Stellarators, on the other hand, have no plasma current. Solid or liquid objects falling into them cause a “radiative collapse”: the stored energy is lost in few ms in the form of a big flash of light. Massless photons cause practically no damage to the device. Nevertheless, the event is still a nuisance, because the plasma needs to be re-initiated and brought to fusion-relevant densities and temperatures again, which takes several seconds. During that time there is no electricity black-out because thermal power plants have a “thermal inertia” and keep producing electricity even if the heat source stops for several seconds.
-
We continuously reduce the frequency of these events and are currently at approximately one droplet per minute in a reduced-scale, cylindrical prototype.
-
The ‘hard’ or essential limit is one droplet every many minutes (10’?) in a full-scale, stellarator reactor - the exact requirement is under discussion with utilities. The reason is that a droplet causes a radiative collapse; after that, the plasma must be re-started and ramped again to fusion densities and temperatures, which takes several seconds. That is, a droplet causes an interruption of less than 1’ in the fusion heat supply. One such interruption every 10’ translates in a duty cycle of 90% or higher. While not taking full advantage of stellarators’ steady-state nature, this duty cycle is still much higher than tokamaks or other fusion devices, and perfectly acceptable for an energy supplier.
It would be even better if droplets were so infrequent that the plasma is only interrupted when planned (for example to remove Helium ashes and impurities and start a new, cleaner plasma) and there are no unplanned interruptions due to droplets. For that, the average time between subsequent droplets falling in the plasma must exceed the plasma-discharge duration, which for stellarators can last hours. Longer than that is possible but unnecessary and counter-productive from an ash, impurity accumulation and other operational standpoints.
-
Droplets form when enough energy is supplied to the fluid to break the surface tension that holds the layer/stream together.
To that end, one must avoid discontinuities in the solid “riverbed” on the side opposite to the plasma, as well as sharp bends and sharp obstacles amidst the flow.
(Magneto)hydrodynamic oscillations, waves, instabilities, and turbulence also perturb the free surface. The good news is that these disturbances are damped by the strong magnetic field and rarely become large enough to break into droplets (“passive stabilization”). Deliberately applied e.m. forces provide additional “active stabilization” or even “feedback stabilization”, in feedback with sensors.
Have a question and don’t see it here?
Send it to contact@renfusion.eu. FAQs will be answered here, less frequent questions will be answered by individual e-mails.